Properties of Metals
DOE-HDBK-1017/1-93
APPENDIX A
For example, if LiD salt is placed in contact with the surface of a stainless steel specimen, the
oxide is reduced, allowing increased permeation. If a metal undergoes surface oxidation in the
presence of steam, permeability decreases as oxidation proceeds.
The mechanical integrity of nonhydriding metals in the presence of tritium is excellent because
the electron bands carry away the energy of colliding beta particles without disrupting the metal
structure or bonding. These metals form the most common class of tritium containment
structural materials. They generally include 304L, 316L, 321, 21-6-9, and Nitronic stainless
steels, as well as copper and aluminum. Inconel, Ni-Cr alloys, and 400-series stainless steels
are generally not chosen because of corrosion or hydrogen embrittlement sensitivity. At high
pressures of tritium gas, however, classical hydrogen embrittlement, as well as helium-3
embrittlement, can occur in accepted materials. For example, for 304L stainless steel samples
exposed to 9 kpsi of tritium at 423 K for 6 months and then aged 1.5 years, fracture toughness
decreased by a factor of 6. Of this, a factor of two could be attributed to helium-3 alone.
Substantially different fracture modes are observed between aged tritium-loaded and unloaded
steel specimens. Helium-3 is vastly less soluble in metals than is hydrogen (tritium); helium
pockets (bubbles) form with high internal pressures. Hydrogen embrittlement also contributes
to this effect.
Permeative escape rates of tritium through nonhydriding metals are generally acceptable at
temperatures below 100C to 300C and for thicknesses of 0.1 cm or more. For 304 stainless
steel 0.3 cm thick with a 1000-cm2 surface area exposed on one side to tritium gas of 1 atm
pressure at 300 K, the permeability is 1.6 x 10-4 Ci/day (t0.9 = 7 hours). The temperature
dependence of permeation is often astounding.
Cross-contamination between nonhydriding metals and tritium does occur often enough to be
troublesome. Oxide layers on metals often contain hydrogen and are further covered with a thin
adsorbed carbonaceous film when originally grown in room air. Upon exposure to such a
surface, tritium gas may become contaminated over hours or days with hundreds to thousands
of parts per million of protium (as HT) and methane (as CT4) as the surface layers are
radiolyzed, exchanged, and contaminated by the material. Because diffusion of tritium in the
bulk material is usually slow at room temperature, the extent of surface oxide contamination may
greatly surpass the bulk contamination of a component. Cross-contamination can be minimized
by minimizing material surface areas, choosing an impermeable material with a thin or
nonexistent oxide layer, and maintaining cleanliness.
Tritium present in an oxide layer can be removed by acid dissolution of the oxide or more gently
by isotopic exchange with normal water or activated hydrogen gas (plasma). Because diffusion
of oxide- or bulk-dissolved tritium back to the surface of a material undergoing decontamination
is often slow, exchange at an elevated temperature may be advantageous.
Rev. 0-A
Page A-3
MS-02
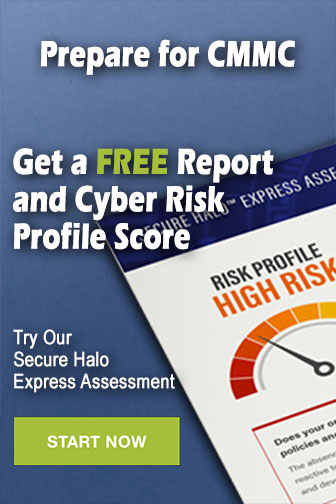